Astronomers have unveiled the first image of the supermassive black hole at the centre of our own Milky Way galaxy. This result adds overwhelming evidence – over length scales comparable with the event horizon – that the object was indeed a black hole and yielded valuable clues about the workings of such giants, which are thought to reside at the centre of most galaxies. The image, in unprecedented resolution, was produced by Event Horizon Telescope (EHT), a global scientific collaboration, where scientists funded by the European Research Council, through BlackHoleCam project, have played a key role.
The breakthrough follows the EHT collaboration’s 2019 release of the first image of a black hole, called M87*, at the centre of the more distant Messier 87 galaxy. The new image is a long-anticipated look at the massive object that sits at the very centre of our own galaxy. Scientists had previously seen stars orbiting around something invisible, ultra-compact, and very massive at the centre of the Milky Way. This shows beyond reasonable doubt that this object known as Sagittarius A* is a black hole, and today’s image provides the first direct visual evidence of it.
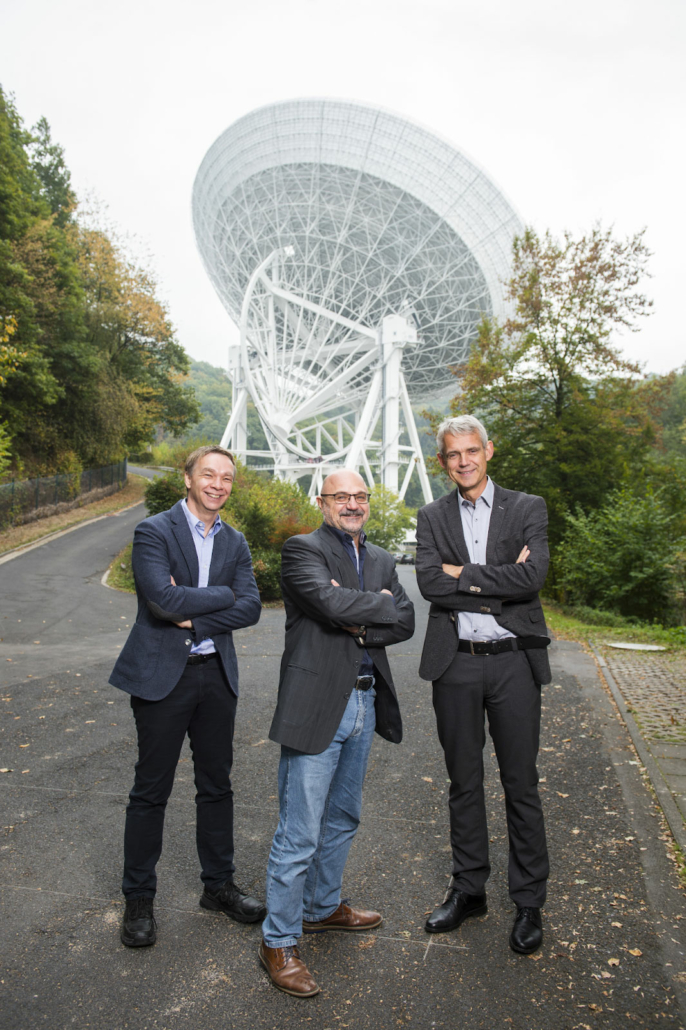
“The idea that our own Milky Way may harbour a supermassive black hole always intrigued and motivated me. Now we can finally see that it is really there. This is a lifelong dream come true,” said Heino Falcke Professor of Astroparticle Physics and Radio Astronomy at Radboud University in Nijmegen, Netherlands.
Professor Falcke together with Professors Michael Kramer from the Max Planck Institute for Radioastronomy, and Luciano Rezzolla from Goethe University Frankfurt, and their teams of postdocs and PhD students and research staff, led BlackHoleCam. The project, proposed in 2013 and supported with an ERC Synergy Grant from 2014 to 2020, aimed not only to produce the first-ever image of a black hole, but also “turn our Galactic Centre into a fundamental-physics laboratory to measure the fabric of space and time with unprecedented precision.” Now these objectives have been fully accomplished.
Although we cannot see the event horizon of the black hole because it is completely dark, hot and glowing gas around it reveals a telltale signature: a dark central region, called a “shadow”, surrounded by a bright ring-like structure. The new view captures light bent by the powerful gravity of the black hole, which is four million times more massive than our Sun. The EHT team’s results are being published today in a special issue of The Astrophysical Journal Letters.
“For me the image of Sgr A* is even more exciting than our first black hole image of M87,” said Professor Kramer. “For Sgr A*, we already knew the mass and distance of the black hole from other measurements. This allows the prediction of the size of the shadow, assuming the general relativity is correct, which we can now compare with the observations. In other words, we can do an exciting test of Einstein’s theory! He wins again!”
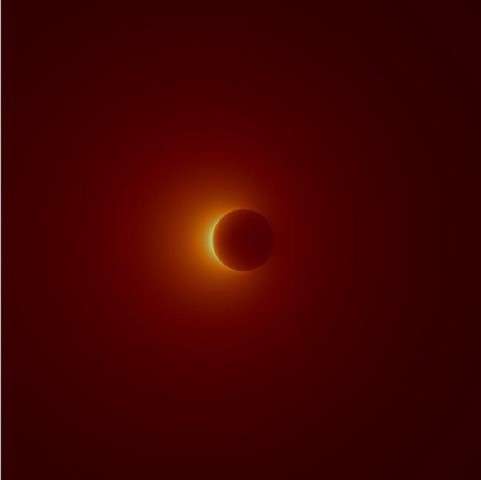
Because the black hole is about 27,000 light-years away from Earth, it appears to us to have about the same size in the sky as a donut placed on the Moon. To image it, the team created the powerful EHT, which linked together eight existing radio observatories across the planet to form a single “Earth-sized” virtual telescope. The EHT observed Sgr A* on multiple nights, collecting data for many hours in a row, similar to using a long exposure time on a camera.
The two black holes that so far have been imaged – M87* and Sgr A* – look remarkably similar, even though our galaxy’s black hole is more than a thousand times smaller and less massive than the colossus pictured in 2019 and the physical conditions very different.
Piecing together the image of Sgr A* – even though it is much closer to us – was considerably more difficult because of the variations in the data. In comparison, M87* was an easier, steadier target, with nearly all images looking the same, that was not the case for Sgr A*. The image of the Sgr A* black hole is an average of the different images the team extracted, finally revealing the giant lurking at the centre of our galaxy for the first time.
“Despite all the experience with M87*, imaging Sgr A* has not been a stroll in the park. The rapid variability in the emission and a much more lively environment at the centre of the galaxy has forced us to develop new techniques for the analysis of the data and of the numerical simulations, said Professor Rezzolla. The end result is that we now have much more confidence on how black holes behave and can discard a number of theoretical models that do not fit observations.”
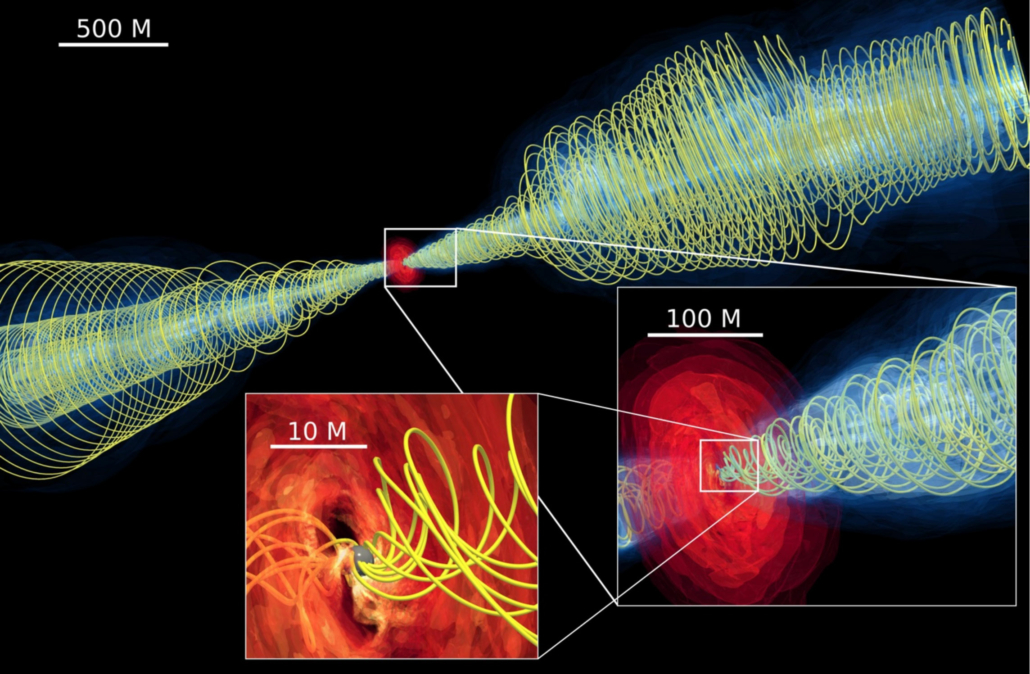
The effort was made possible through the ingenuity of more than 300 researchers from 80 institutes around the world that together make up the EHT Collaboration. Along with the US’s National Science Foundation, the European Research Council (ERC) has provided crucial support to the EHT though the €14 million BlackHoleCam project. Over the past two decades the EU also supported the development and upgrading of the large telescope infrastructure essential to the success of the EHT project, investing more than €30 million in RadioNet.
In addition to developing complex tools to overcome the challenges of imaging Sgr A*, the EHT team worked rigorously for five years, using supercomputers (some of which were fully funded by the ERC) to combine and analyse their data, all while compiling an unprecedented library of simulated black holes to compare with the observations. Scientists are particularly excited to finally have images of two black holes of very different sizes, which offers the opportunity to understand how they compare and contrast. They have also begun to use the new data to test theories and models of how gas behaves around supermassive black holes, and whether alternatives to black holes are compatible with the data. This process is not yet fully understood, but is thought to play a key role in shaping the formation and evolution of galaxies.
Related pages:
https://erc.europa.eu/news-events/magazine/Behind-the-scene-with-blackhole-scientists
https://erc.europa.eu/projects-figures/stories/astronomers-reveal-first-ever-image-black-hole
https://erc.europa.eu/news/eu-funded-scientists-unveil-first-ever-image-black-hole
https://www.youtube.com/watch?v=Dr20f19czeE
Other ERC-funded research on black holes:
- Launching, propagation and emission of relativistic jets from binary mergers and across mass scales (JETSET) https://cordis.europa.eu/project/id/884631
- Mapping Magnetic Fields with INterferometry Down to Event hoRizon Scales (M2FINDERS) https://cordis.europa.eu/project/id/101018682